Plant sensors could act as an early warning system for farmers
Using a pair of sensors made from carbon nanotubes, researchers from MIT and the Singapore-MIT Alliance for Research and Technology (SMART) have discovered signals that reveal when plans are experiencing stresses such as heat, light or attack from insects or bacteria.
The sensors detect two signaling molecules that plants use to coordinate their response to stress: hydrogen peroxide and salicylic acid (a molecule similar to aspirin). The researchers found that plants produce these molecules at different timepoints for each type of stress, creating distinctive patterns that could serve as an early warning system.
Farmers could use these sensors to monitor potential threats to their crops, allowing them to intervene before the crops are lost, the researchers say.
“What we found is that these two sensors together can tell the user exactly what kind of stress the plant is undergoing. Inside the plant, in real time, you get chemical changes that rise and fall, and each one serves as a fingerprint of a different stress,” said senior author Michael Strano of MIT. The research was published in Nature Communications.
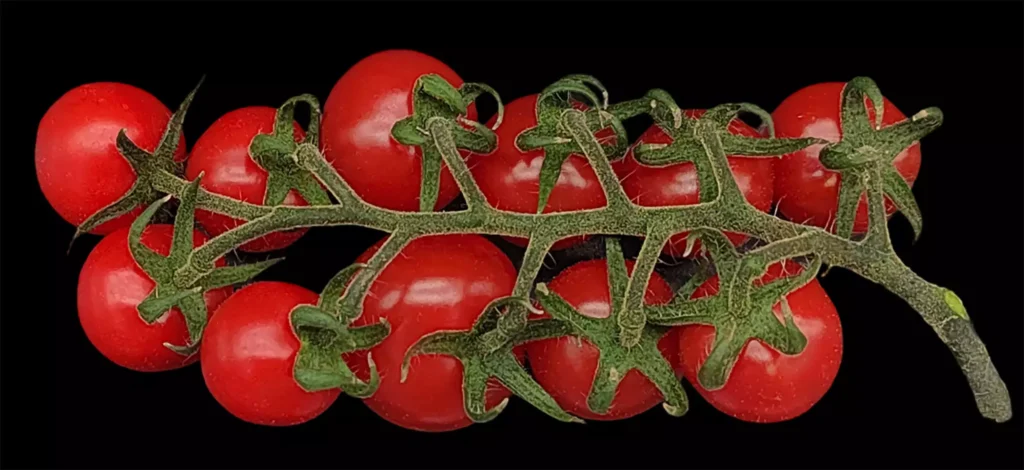
Plants respond to different kinds of stress in different ways. In 2020, Strano’s lab developed a sensor that can detect hydrogen peroxide, which plant cells use as a distress signal when they are under attack from insects or encounter other stresses such as bacterial infection or too much light.
These sensors consist of tiny carbon nanotubes wrapped in polymers. By changing the three-dimensional structure of the polymers, the sensors can be tailored to detect different molecules, giving off a fluorescent signal when the target is present. For the new study, the researchers used this approach to develop a sensor that can detect salicylic acid, a molecule that is involved in regulating many aspects of plant growth, development, and response to stress.
To embed the nanosensors into plants, the researchers dissolve them in a solution, which is then applied to the underside of a plant leaf. The sensors can enter leaves through pores called stomata and take up residence in the mesophyll — the layer where most photosynthesis takes place. When a sensor is activated, the signal can be easily detecting using an infrared camera.
In this study, the researchers applied the sensors for hydrogen peroxide and salicylic acid to bok choy. Then, they exposed the plants to four different types of stress — heat, intense light, insect bites and bacterial infection — and found that the plants generated distinctive responses to each type of stress.
Each type of stress led the plants to produce hydrogen peroxide within minutes, reaching maximum levels within an hour and then returning to normal. Heat, light and bacterial infection all provoked salicylic acid production within two hours of the stimulus, but at distinct time points. Insect bites did not stimulate salicylic acid production at all.
The findings represent a “language” that plants use to coordinate their response to stress, Strano says. The hydrogen peroxide and salicylic acid waves trigger additional responses that help a plant survive whatever type of stress it’s facing.
For a stress such as an insect bite, this response includes the production of chemical compounds that insects don’t like, driving them away from the plant. Salicylic acid and hydrogen peroxide can also activate signaling pathways that turn on the production of proteins that help plants respond to heat and other stresses.
This technique is the first that can obtain real-time information from a plant, and the only one that can be applied to nearly any plant. Most existing sensors consist of fluorescent proteins that must be genetically engineered into a specific type of plant, such as tobacco or the common experimental plant Arabidopsis thaliana, and can’t be universally applied.
The researchers are now adapting these sensors to create sentinel plants that could be monitored to give farmers a much earlier warning when their crops are under stress. When plants don’t have enough water, for example, they eventually begin to turn brown, but by the time that happens, it’s usually too late to intervene.
This technology could also be used to develop systems that not only sense when plants are in distress but would also trigger a response such as altering the temperature or the amount of light in a greenhouse.
The researchers are also developing sensors that could be used to detect other plant signaling molecules, in hopes of learning more about their responses to stress and other stimuli.
Barley plants fine-tune their root microbial communities through sugary secretions
Different types of barley recruit distinct communities of soil microbes to grow around their roots by releasing a custom mix of sugars and other compounds, according to a new study published in PLOS Biology.
Beneficial soil microbes that live on or around plant roots can provide nutrition, help the plant withstand stress and protect it from pathogenic microbes. In return, the plant secretes a portion of the sugars it makes through photosynthesis, along with amino acids and other metabolites, into the surrounding environment. The composition of soil microbes that a plant attracts can vary widely, depending on what kinds of compounds the plant provides.
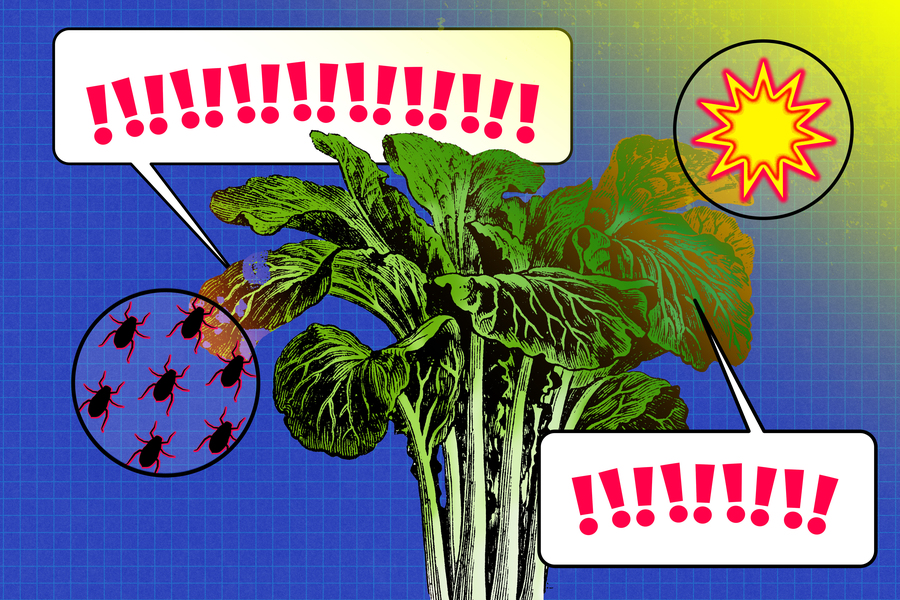
In the new study, researchers took a closer look at the relationship between a plant’s genetics and its root microbes. They analyzed the microbiomes from two types of barley plants — a modern type named Tipple, and a traditional one, named Chevallier.
The two barley types each had an overlapping core group of microbes associated with their roots, but with some distinct differences in the overall communities. One common group of root microbes — the Pseudomonas genus — was especially abundant around Tipple roots. The researchers discovered that this is because Tipple plants secrete large amounts of sugars called hexoses (such as glucose or fructose), which Pseudomonas especially like to eat.
They also observed differences in gene activity between the two barley types that helped explain the variation in their root communities. When researchers tried to swap the microbial communities between the two plant types, each barley variety grew better when exposed to its original community.
The new findings suggest that plants can fine-tune the population of microbes colonizing its root zone, which can have important outcomes for plant health.
The spiciness of mustard may depend on soil microbes
Wine connoisseurs firmly believe that the soil in which grapes are grown determines how it tastes. Can microbes in the soil also contribute to taste?
In a recent study published in New Phytologist, researchers from the university of Colorado Boulder ran one of the first experiments to figure out if soil microorganisms like bacteria and fungi influence the flavor of a crop. Their target: the spiciness of mustard seeds.
Previous research has confirmed that soil properties influence plant characteristics like growth, seasonal cycles, disease resistance and nutrient absorption. What remains a mystery is whether or not soil microbes influence a plant’s flavor. Testing for this is difficult — past studies have surveyed plants grown in different locations and regions, making it tricky to isolate the role of microbes alone.
The researchers took a unique approach: they used a greenhouse study and grew mustard plants while inoculating the plants with a liquid inoculum of microbes from soils in aspen groves, fields of sagebrush, ponderosa pine forests and agricultural pastures, all in Colorado. The potting soil, temperature, watering, and nutrients were held constant — only the microbes varied.
They chose mustard because it’s easy to grow, and its spicy and bitter flavors come from glucosinolates, sulfur-containing chemicals that help brassica plants defend against insects and pests.
After harvesting the mustard seeds, the researchers tested for spiciness by measuring glucosinolate concentrations in the mustard seeds to observe different flavor chemicals.
They concluded that there is some evidence of a relationship between microbes and the spiciness of mustard, but why mustard gets spicier in response to certain microbes remains unknown. However, the work is an important contribution as it adds to our understanding of the myriad ways soil microbes can influence plants.
Probiotic feed additive boosts growth, health in poultry in place of antibiotics
Antimicrobial resistance is an increasingly serious threat for public health, and the use of antimicrobials in livestock feed has been a major contributing factor in the emergence and spread of antimicrobial resistance to many drugs, according to the U.S. National Institutes of Health.
The growing need for antibiotic-free products has challenged producers to decrease or completely stop using antimicrobials as feed supplements in the diet of broiler chickens to improve feed efficiency, growth rate and intestinal health. A Penn State research team recently conducted a study of natural feed additives that are promising alternatives to antimicrobial growth promoters.
In findings published in Poultry Science, the researchers characterized the effects of a probiotic and a blend of essential oils on broilers’ growth and gut health. The team found that supplementing the diet of young chicks with a probiotic over 21 days significantly boosted the abundance of beneficial intestinal microorganisms.
A total of 320 one-day-old chicks were raised for 21 days in 32 randomly allocated cages. Treatments consisted of four experimental diets: a standard diet, and a standard diet mixed with the antibiotic bacitracin methylene disalicylate, or an essential oils blend of oregano oil, rosemary and red pepper, or the probiotic Bacillus subtilis.
Probiotics are live microorganisms that, when administered in adequate amounts, confer a health benefit on the host by supporting a more diverse population of beneficial bacteria in the digestive tract. Essential oils are plant-derived extracts that possess various antioxidant, immune-modulating, antimicrobial properties and the ability to change the microbiome — in this case, the community of microorganisms living in the chicks’ intestines.
The researchers individually weighed all broiler chickens on day one and then at the end of each dietary phase on day 10 and day 21. The feed consumed per pen was monitored at the end of each growth phase. The team also calculated the daily body weight gain average, feed intake and feed conversion ratio of feed consumed divided by weight gain at three periods: the starter phase of one to 10 days, the grower phase of 11 to 21days and the total period of one to 21 days.
The team collected excreta samples daily during the entire experimental period and analyzed DNA to identify bacteria present. Across all time points, supplementing chicken diets with the probiotic or the antibiotic significantly changed the relative abundance of bacterial strains compared to the standard diet. However, there were no microorganisms affected by essential oils compared to the standard diet.
Like dad and like mom … all in one plant
In a new study from the Max Planck Institute for Plant Breeding Research, scientists established a system to generate clonal sex cells in tomato plants and used them to design the genomes of offspring. The fertilization of a clonal egg from one parent by a clonal sperm from another parent led to plants containing the complete genetic information of both parents. The study was published in Nature Genetics.
Hybrid seeds, combining two different parent lines with specific favorable traits, are popular in agriculture as they give rise to robust crops with enhanced productivity; they have been utilized by farmers for over a hundred years.
The increased performance of hybrids is generally known as hybrid vigor, or heterosis, and has been observed in many different plant (and animal) species. However, the heterosis effect no longer persists in the subsequent generations of these hybrids due to the segregation of genetic information. Thus, new hybrid seeds need to be produced every year — a labor-intensive and expensive endeavor that doesn’t work well for every crop.
So, how can the beneficial traits, encoded in the genes of hybrid plants, be transferred to the next generation?
Typically, our genetic material undergoes reshuffling during meiosis — a crucial cell division occurring in all sexually reproducing organisms. This reshuffling, due to random segregation of chromosomes and meiotic recombination, is important in generating novel and beneficial genetic configurations in natural populations and during breeding.
However, when it comes to plant breeding, once you have a great combination, you want to keep it and not lose it by reshuffling the genes again. Having a system that bypasses meiosis and would result in sex cells (egg and sperm) that are genetically identical to the parents could have several applications.
In this study, lead researcher Charles Underwood and his team established a system in which they replaced meiosis with mitosis, a simple cell division, in the most popular vegetable crop plant, the cultivated tomato. In the so-called MiMe system (Mitosis instead of Meiosis) the cell division mimics a mitosis, thus sidestepping genetic recombination and segregation, and produces sex cells that are exact clones of the parent plant. The concept of the MiMe system has previously been established in Arabidopsis and rice.
A breakthrough aspect of the new study is that for the first time the researchers harnessed the clonal sex cells to engineer offspring through a process they call “polyploid genome design.”
Usually, sex cells have a halved chromosome set (in humans, 46 chromosomes reduces to 23; in tomato 24 chromosomes reduces to 12). However, the MiMe sex cells are clonal, and therefore this halving of the chromosome set does not happen. Underwood and his team performed crosses that meant that the clonal egg from one MiMe tomato plant was fertilized by a clonal sperm from another MiMe tomato plant. The resulting tomato plants contained the complete genetic repertoire of both parents — and are thereby made up of 48 chromosomes. Hence, all favorable characteristics from both hybrid parents are consolidated — by design — in one novel tomato plant.
Because of the close genetic relationship between tomatoes and potatoes, the team believes that the system described in this study can be easily adapted for use in potato, the world’s fifth most valuable crop plant, and potentially other crop species.
Editor’s note: Count us skeptical that this attempt to improve on the design of nature will lead to only beneficial outcomes.
Correction: In the May Eco-graphic, in reference to the prevention of apple scab, the article should have read “it’s because I plant a wormwood plant every 50 feet through my orchard.” Our apologies. |