Farming with the provision and utilization of nature’s resources
Nature provides for natural forests, grasslands, and prairies in such abundance. Can growers emulate this process — farming in the image of nature — as the basis for a new type of agriculture?
All living entities, from the most minute microbe to humans, contain the following basic elements: C, H, O, N, P, K, Ca, Mg, S, Cl, Na, Fe, Mn, Cu, Zn, B, Mo and Ni. These are the essential macro- and micronutrients required for plant growth. Many scientists also include the following elements: Se, Cr, Co, Ti, Sr, Sn, As, Ba, Ag and Zr. Some highly respected scientists include all the elements from the periodic table!
Where do these elemental building blocks originate from in nature’s supply model?
Origin of the Elements
Oxygen (65 percent), hydrogen (10), carbon (18) and nitrogen (3) make up about 96 percent of the total minerals contained in living cells. Hydrogen and oxygen emanate from the atmosphere — as water vapor and rain (H2O) and carbon (CO2). Nitrogen makes up 78 percent of the atmosphere.
The final 4 percent of cells’ make-up originates from the soil. This includes the following macro-, micro- and nano-nutrients:
Macro: P, K, Ca, Mg, S, Cl
Micro: Na, Fe, Mn, Cu, Zn, B, Mo, Ni, Se
Nano: Cr, Co, Ti, Sr, Sn, As, Ba, Ag, Zr
If we analyze soils for the total mineral content using a “total nutrient digestion” test, we will find out not only the nutrients in suspension or those adhered to the cation exchange points — as in a soil chemical analysis. We will get a very different picture of the actual mineral contents — not necessarily those that are available, but those that are potentially available.
Figure 1 shows results for total nutrient tests from Dr. Sparks from the University of Delaware — estimating average soils worldwide — and work in progress by Mr. Gabe Brown for General Mills in North Dakota and Canada.
Nature has at its disposal a vast amount of mineral nutrients in unavailable solid-state form — as well as atmospheric minerals in the gas form.
How does nature put its growth model together utilizing the mineral nutrients from these unavailable forms? With life — microbial life!
It is important to understand that all living species have an interdependence with microbial life. A human being has about 100 trillion cells, of which 90 trillion are microbes living in mutual association with the human cells. This mutual interdependence forms the basis for the maintenance of plant, animal and human health.
Describing Nature’s Model
Carbon, Hydrogen and Oxygen
The process of photosynthesis — the absorption of CO2 using sunlight energy capture — provides the carbon and part of the oxygen that plants turn into carbohydrates. The absorption of water through roots delivers the hydrogen and the other part of the oxygen. During photosynthesis, two thirds of the oxygen returns to the atmosphere.
Nitrogen
Nitrogen is probably the most difficult element to understand because it resides in the atmosphere as a gas that needs to be transformed into a solid-state organic or inorganic form for plant roots to absorb. In nature’s model, nitrogen is captured by soil microorganisms and made available to plants. In conventional agriculture it is also captured from the atmosphere but is stored and applied in inorganic mineral fertilizer forms utilizing the Haber-Bosch process.
The average American soil contains around 2,000 to 5,000 ppm of organic nitrogen (3,200 to 8,000 pounds organic nitrogen per acre-ft), which is derived from decaying organic material and microbial biomass — of which about 40 to 60 pounds, on average, is plant available.
Atmospheric nitrogen gas is fixed into ammonia through a reductive biochemical process in the presence of the enzyme nitrogenase, facilitated by a specialized group of bacteria known as diazotrophs. This process is the same as that facilitated through legume-associated Rhizobium bacteria or through other nitrogen-fixing bacteria that live in association with grasses or other plant species. The next step is the binding of the ammonia with organic acids to form amino acids, which are the building blocks of proteins. These amino acids are provided to the nutrient pool in the rhizosphere for plant or bacterial food and are referred to as “water-extractable organic nitrogen.” Plants absorb these amino acids as the precursor building blocks of proteins, as do microbes.
There is strong evidence that the demand for nitrogen and for all other required nutrients within a plant is regulated through signaling systems between plant, bacteria and fungi. Signals from the plant requiring a certain quantity of nitrogen are sent through the roots to the nitrogen-fixing bacteria within the rhizosphere. They multiply into a quorum and provide the nitrogen-fixing service. Once the tasks have been completed, the specific food source is withdrawn by the plant, and the excessive microbial population becomes a food source for protozoa, nematodes and other microorganisms higher up in the predation order. With this predation, more nutrients of all kinds are released into the nutrient pool around the root for consumption by the plant. There are also new bursts of diverse microbial life called upon to fulfill other soil health and plant protection tasks.
It therefore becomes obvious that the diversity of the microbial communities within a natural system must be extremely high. The maintenance of these communities can only be secured through a diversity of plants, with each plant species responsible for the feeding of a different group, due to their differing root-microbial associative characteristics.
Other Soil Minerals
A fungal group known as mycorrhizae performs a very important function in nature. There are presently about 260 known mycorrhizae species across seven families, and certainly a large number has not yet been identified. They are known to fulfill their function in association with bacteria known as mycorrhizae helper bacteria. These mycorrhizae species are completely dependent on host plants for their food through root exudates. One group, known as vesicular arbuscular mycorrhizae, form root-associated appendices called arbuscules in host plant roots, while another group — ecto-mycorrhizae — colonize the outside of the root tip within the rhizosphere.
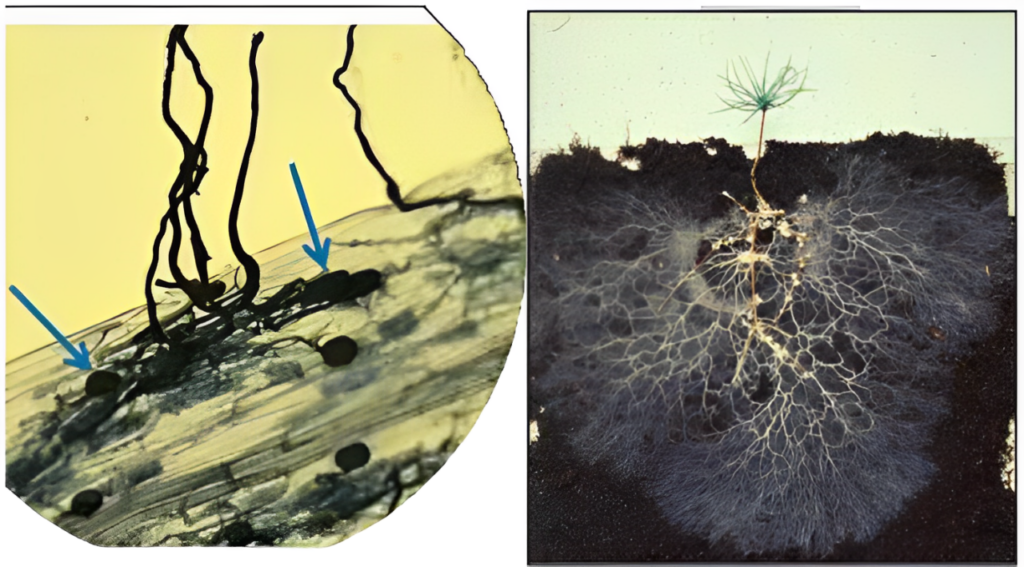
The fungal hyphae of the mycorrhizae serve as root extensions, adding miles of additional root transportation networks to the host plant. They are a fraction the thickness of a root hair and can penetrate hairline cracks to obtain nutrients and water for the host plant. Within these micropores, water is still present but not accessible to the roots. Even when the plant’s wilting point has technically been reached, mycorrhizae hyphae can still extract water for the host plant.
Connectivity between Plant and Soil Minerals
Mycorrhizae are nature’s most important tool for providing minerals to the host plant. This connectivity — also known as the plant-root-soil internet highway — conveys inter-plant messages between plants and roots and also serves as the conduit for mineral supplies in an orderly fashion. The hyphal strands also connect plants within a community of the same and different species with each other, creating an inter-plant communication system. This is a very interesting topic that is presently receiving plenty of research attention — confirming, among other things, that an insect or other attack on one plant is signaled throughout the connected community so that natural defense mechanisms are triggered by the other community members in anticipation of attacks.
The fungal hyphae grow throughout the soil profile, extending many meters beyond the host plant’s root system, attaching to many mineral sites where the different plant-required elements are in abundance — albeit in the solid, insoluble state. Should the host plant require any mineral to which the mycorrhizae has an attachment, a signal for such a request would be transmitted through the fungal communication highway to this site, where the helper bacteria would solubilize the nutrient from the solid state and transport the required quantity back through the fungal hyphae to the root for use by the host plant, where required, in specific growth cycles. Fungal hyphae also excrete oxalic acid — one of the strongest organic acids — that allows the hyphae to also “drill” their way through solid granite, capturing elemental nutrients along the way.
In the process of hyphae extension, an extremely concentrated organic carbon substance with a carbon content of more than 30 percent, known as glomalin, is created. Glomalin is a recalcitrant, extremely stable glycoprotein. As segments of the mycorrhizae hyphae senesce and become dysfunctional, this glomalin is released, binding soil particles and thus improving soil aggregate stability. The mycorrhizal mutualistic association therefore not only provides nutrients to the host plants but also maintains other soil functions, such as infiltration and water holding capacity, through aggregation of soil particles.
The importance of this mycorrhizae-aided nutrient supply system and its connectivity to the soil minerals cannot be over emphasized. It is a system that naturally selects for the required nutrient as well as for the sufficiency thereof.
Plant-root Mycorrhizal Connectivity Effects on Health
In agricultural production systems where this mycorrhizal connectivity is absent, as is the case with many conventional chemical-driven practices, plant roots are dependent on elements in the soluble, plant-available form, with a very limited root surface area. The signaling system is largely impaired, and roots can only absorb minerals when these become available within the vicinity of a root — usually minerals from applied fertilizers or dissolved from the natural soil minerals at appropriate pH levels. The driver of conventional systems — where microbiology is largely absent or not taken into consideration — is therefore pH. The very important but unfamiliar micro- and nano-nutrients required for healthy individuals are therefore not readily available and are thus not absorbed and become deficient within the food supply chain.
The alarming issue is that most corn, wheat, soybeans and other commercially grown crops are selected in breeding programs to accommodate these deficiencies. Breeders mainly selected for yield under higher and higher fertilizer regimes, with narrower and narrower micro- and nano-nutrient requirements. The crops resulting from such breeding programs are therefore less nutrient dense, with more carbohydrate bulk to support yield.
Rhizophagy
Rhizophagy is another natural microbial-aided nutrient supply system very recently described in detail by Dr. James White of Rutgers University. It is a mechanism for the transfer of nutrients from symbiotic microbes (bacteria and fungi) to host plant roots.
In the rhizophagy cycle, microbes alternate between a root-intracellular endophytic phase and a free-living soil phase outside the root. Microbes acquire soil nutrients in the free-living soil phase within the rhizosphere, as described above, and then enter root-tip meristem cells as complete bacteria cells, within the periplasmic spaces between cell walls and plasma membranes. Nutrients from these bacteria cells are then extracted through exposure to host-produced reactive oxygen that degrades the bacterial cell walls.
Dr. White found that the symbiotic microbes initially grow on the rhizoplane in the exudate zone, adjacent to the root meristem, outside the root. Inside the root tip, the bacterial cells within the periplasmic spaces of the root cells convert to cell-wall-less protoplast forms because of the reactive oxygen, which degrades their cell walls, effectively extracting nutrients from the microbes. Surviving bacteria in root epidermal cells trigger root hair elongation, and as hairs elongate, bacteria exit at the hair tips, reforming their cell walls and cell shapes as these microbes emerge into the rhizosphere, where they may obtain additional nutrients and then repeat the cycle. The rhizophagy cycle has been found to positively impact N, P and K, as well as most macro- and micronutrients.
Enabling plants to live in symbiotic association with soil microbes — such as in the rhizophagy cycle or via mycorrhizal associations, as well as with other types of microbial endophytic associations — has many benefits to crop plants and should be embraced in crop production practices.
Growers who are aware of how soil biology works can emulate nature’s processes to create successful cropping systems.
Willie Pretorius is a soil health consultant with Ward Laboratories. He has degrees from the Universities of Pretoria and Stellenbosch.
The author would like to thank Dr. David C Johnson, Senior Research Scientist at the Institute for Sustainable Agriculture, and Dr. James F. White, Department of Plant Biology, Rutgers University, for their contributions to this article.
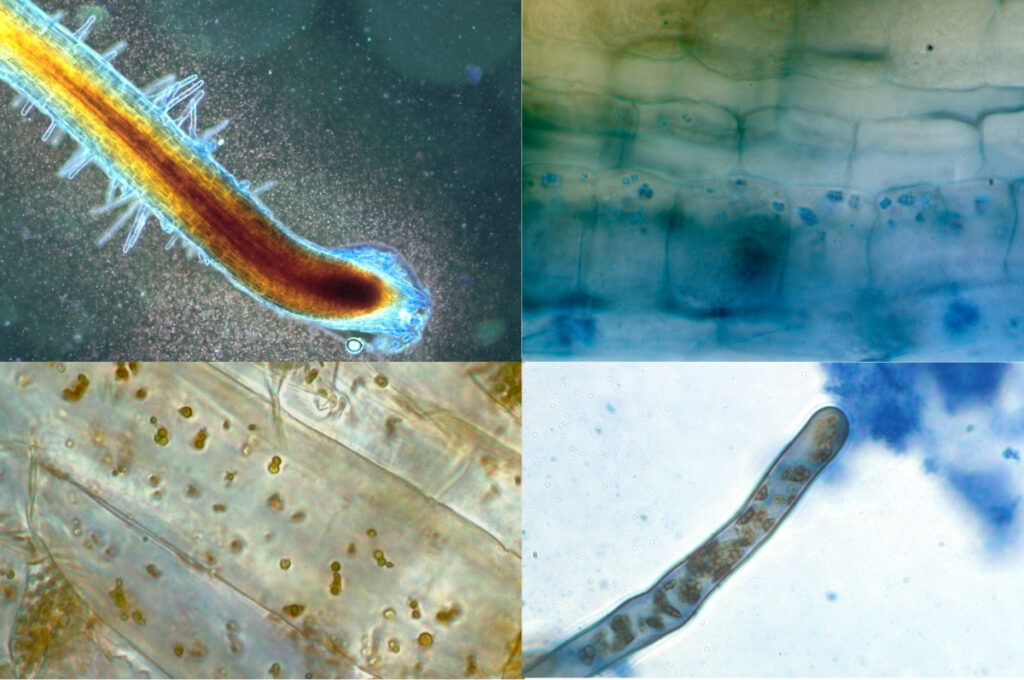
Top right: The root epidermal cells of curly dock (Rumex crispus) showing tetrads of the bacterium Micrococcus luteus within cells.
Bottom left: Root epidermal cells of common reed grass (Phragmites australis) showing bacterial protoplasts replicating and degrading within the cell.
Bottom right: A root hair of a fescue grass showing bacteria within the hair (brown due to hydrogen peroxide around bacteria) and bacteria ejected from the tip in mass (blue staining).