Roots Measure Heat to Adjust Their Growth
Plant roots have their own thermometer to measure the temperature of the soil around them, and they adjust their growth accordingly. A team from Martin Luther University Halle-Wittenberg was able to demonstrate that roots have their own temperature sensing and response system. In a new study in the journal EMBO, the scientists also provide a new explanation for how roots detect and react to higher temperatures. The results could help develop new approaches for plant breeding.
The researchers used climate chambers to investigate how thale cress, cabbage and tomatoes react to rising ambient temperatures, from 20 to 28°C (68 to 82.4 degrees Fahrenheit). “Until now, it was assumed that the plant shoot controlled the process for the entire plant and acted as a long-distance transmitter that signaled to the root that it should alter its growth,” said Professor Marcel Quint.
In one experiment, scientists cut off the shoot of the plants but allowed the roots to continue to grow. “We found that the roots were not affected by this and grew at elevated temperatures in the same way as on plants with intact shoots. The higher temperature stimulated cell division and the roots became significantly longer,” said Quint. The team also used mutant plants whose shoots could no longer detect and respond to higher temperatures. Those were grafted onto roots without this defect. Here, too, the roots were able to react to the heat in the soil, even though the shoot did nothing.
The researchers found in all of their experiments that root cells increased the production of the growth hormone auxin, which was then transported to the root tips. There, it stimulated cell division and enabled the roots to reach farther down into the soil. “As heat and drought usually occur in tandem, it makes sense for the plants to tap into deeper and cooler soil layers that contain water,” Quint explains.
Scientists have understood how plant shoots react to higher temperatures for some time. Their cells also produce more auxin, but the aboveground plant reacts differently than its roots. The cells in the shoot stretch, the stalk grows taller, and the leaves become narrower and grow farther apart.
Related to this article, see also the new paper “Plant Diversity Stabilizes Soil Temperature” in the journal bioRxiv. “Using 18 years of a continuous dataset with a resolution of 1 minute (∼795,312,000 individual measurements) from a large-scale grassland biodiversity experiment, we show that plant diversity buffers soil temperature throughout the year. Plant diversity helped to prevent soil heating in hot weather, and cooling in cold weather. Moreover, this effect of plant diversity increased over the 18-year observation period with the aging of experimental communities and was even stronger under extreme conditions, i.e., on hot days or in dry years. Using structural equation modelling, we found that plant diversity stabilized soil temperature by increasing soil organic carbon concentrations and, to a lesser extent, by increasing the plant leaf area index. We suggest that the diversity-induced stabilization of soil temperature may help to mitigate the negative effects of extreme climatic events such as soil carbon release, thus slow global warming.” |
Building Models to Predict Interactions in Plant Microbiomes
Plants, animals, and humans are all home to numerous microorganisms such as bacteria and fungi. These form complex communities that have a profound impact on the health of their host.
Plants host to microbial communities on their roots and leaves. These communities can promote growth and keep off harmful bacteria. Plant microbiomes therefore have the potential to make agriculture more sustainable. However, we currently only have a rudimentary understanding of the interspecies interactions that shape these microbial communities.
Why is it that these communities tend to be populated only by certain kinds of microbes and not others? “We already knew that leaf microbiomes weren’t just some random collections of microbes,” says Julia Vorholt, Professor of Microbiology at ETH Zurich. “But the rules that determine how these communities form and what mechanisms shape their makeup remained to be found.”
Now, a team of researchers at ETH Zurich has identified just such an organizing principle for the bacteria that live on the leaves of the model plant Arabidopsis thaliana (thale cress). The researchers have developed a set of models that use the nutrient preferences and metabolic abilities of individual bacterial strains to predict how these leaf surface microbes compete or cooperate with each other, thereby helping us better understand the nature of the resulting microbiome. The research team’s study has been published in the latest issue of the journal Science.
Vorholt’s group had already shown that the microbial communities found on plant leaves are remarkably similar. “The consistent composition of these communities points to an underlying mechanism that controls how the leaf microbiome is created,” Vorholt says.
Martin Schäfer, a co-lead author on the study, explained that “since all bacteria ultimately depend on organic molecules as food, we asked whether we could predict the way they interact by knowing which food molecules they can metabolize.” The guiding question posed by the team was whether the metabolic capabilities of different bacteria can explain how the leaf microbiome takes shape.
The researchers began by testing the ability of more than 200 representative strains of bacteria from Arabidopsis thaliana leaves to grow using 45 different carbon sources. Using these carbon profiles, they determined that there was extensive overlap between the strains’ food niches. This indicates that there is fierce competition for resources.
The researchers then used these carbon profiles to build a set of reliable metabolic models for all bacterial strains and simulated interactions between more than 17,500 pairs of bacteria. Consistent with the extensive overlap in food niches, the simulations showed a marked dominance of negative interactions: when competition causes the population of at least one of the two strains to decrease.
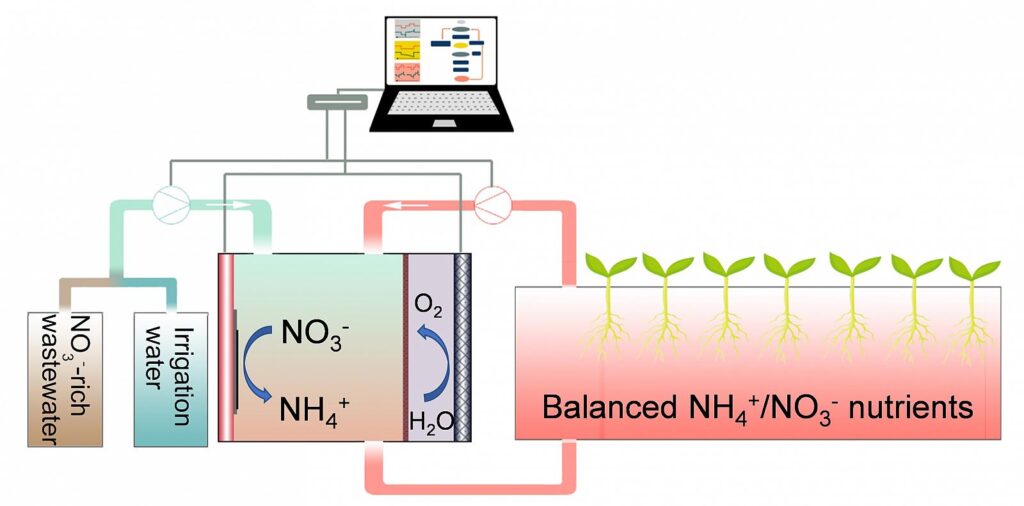
Despite this prevalence of competition, the metabolic models also predicted positive interactions. A closer analysis revealed that these cooperative interactions can be traced back to the exchange of organic and amino acids. The team carried out plant experiments to test the models’ predictions and were able to confirm them to an accuracy of 89 percent.
“What’s great about our models is that they also work in reverse,” Vorholt said, “in that they can be used to identify mechanisms that trigger certain interaction patterns.” This paves the way for targeted microbiome design, which is a key prerequisite for downstream applications in agriculture.
Currently, seed companies and agricultural chemical producers use a process of trial and error to search for microbes that sustainably support crop protection. The team’s findings are therefore relevant not only for fundamental research, but also for applications in microbiome design for agriculture.
Smart farming platform improves crop yields, minimizes pollution
A new farming system developed by researchers at the University of Texas at Austin aims to solve one of the biggest problems in modern agriculture: the overuse of fertilizers to improve crop yields and the resulting chemical runoff that pollutes the world’s air and water.
The smart farming system uses a copper-based hydrogel that captures excess nitrate waste from fertilizer runoff and transforms it into ammonia — a critical element in fertilizers — that can then be reused. In tests, the system had the ability to match or increase crop yields over traditional methods while also minimizing environmental impacts.
“We designed this system and showed that it can grow the same or more crops without overusing nitrogen, which can contaminate groundwater and lead to harmful greenhouse gasses,” said Guihua Yu, a professor of materials science at UTA.
The “smart farming system” produced wheat and rice plants that grew taller with bigger leaves, compared with other methods, with less nitrogen runoff.
The study, published in the Proceedings of the National Academy of Sciences, shows that the copper-based gel film not only produces ammonia from nitrate waste but also senses nitrogen levels in the soil. This detection capability helps determine the optimal time to drain nitrate, a nitrogen compound that is important for plant growth but can be a pollutant, from the soil to convert to ammonia, keeping it from escaping and contaminating the surrounding environment.
As part of the project, the researchers worked with agricultural experts to compare their work to traditional farming methods. The smart farming system produced wheat and rice plants that grew taller with bigger leaves, compared with other methods, with less nitrogen runoff.
In addition to environmental impacts, excess use of nitrogen fertilizers can also stunt the growth of crops, defeating their purpose of improving production. By simultaneously producing ammonia and monitoring nitrogen levels, this new technology improves crop growth by helping plants take in and use nitrogen more efficiently.
Farming isn’t the only industry that creates significant nitrogen pollution. Industrial and municipal wastewater often features high levels of nitrate because of production of electronics, food processing, textile manufacturing and more.

“We need to feed our growing population, but we also need to protect our water and air,” Yu said. “Finding ways to capture and recycle nitrate-heavy wastewater could have tremendous benefits across the board.”
Scientists Build a Healthy Dietary Pattern Using Ultra-Processed Foods
Editor’s note: We bring you these reports on new scientific and technological discoveries every month because they truly can be important in helping us understand how the natural world works and how that understanding can inform better farming practices. However, we present this piece as an example of why we can’t accept everything commonly called “science.”
Scientists at the USDA Agricultural Research Service’s (ARS) Grand Forks Human Nutrition Research Center led a study that demonstrates it is possible to build a healthy diet with 91 percent of the calories coming from ultra-processed foods (as classified using the NOVA scale) while still following the recommendations from the 2020-2025 Dietary Guidelines for Americans (DGA). The study highlights the versatility of using DGA recommendations in constructing healthy menus.
“The study is a proof-of-concept that shows a more balanced view of healthy eating patterns, where using ultra-processed foods can be an option,” said ARS Research Nutritionist Julie Hess. “According to current dietary recommendations, the nutrient content of a food and its place in a food group are more important than the extent to which a food was processed.”
In the study, scientists used the NOVA scale to determine which foods to classify as ultra-processed. The NOVA scale first appeared in literature in 2009 and is the most commonly used scale in nutrition science to classify foods by degree of processing. According to the NOVA scale, foods can be classified into four groups depending on their degree of processing: (1) unprocessed or minimally processed foods; (2) processed culinary ingredients; (2) processed foods; and (4) ultra-processed foods.
To test if ultra-processed foods can be used to build a healthy diet, ARS scientists and collaborators created a menu with breakfast, lunch, dinner and snacks using MyPyramid as a guide for a seven-day, 2,000-calorie food pattern. The menu consisted of foods categorized as ultra-processed by at least two NOVA graders. The foods included in the menu also aligned with 2020 DGA recommendations for servings of groups and subgroups of fruits, vegetables, grains, protein foods and dairy. Scientists selected food products that have lower levels of saturated fats and added sugars while still containing enough micronutrients and macronutrients. Some of the ultra-processed foods used in this menu included canned beans, instant oatmeal, ultra-filtered milk, whole wheat bread and dried fruit.
“We used the Healthy Eating Index to assess the quality of the diet as it aligns with key DGA recommendations,” said Hess. “The menu we developed scored 86 of 100 points on the Healthy Eating Index-2015, meeting most of the thresholds, except for sodium content [exceeded recommendations] and whole grains [below recommendations].”
Scientists will continue researching this concept, understanding that observational research indicates that ultra-processed products could be associated with adverse health outcomes. This research shows that there is a role for a variety of foods when building a healthy diet and that more research is needed in this field, especially intervention studies.Details of the study were published in the Journal of Nutrition.