Improving water quality and quantity through biology
It’s been said, “major on the majors, and minor on the minors.” While opinions may vary as to what is major, I think we can all agree that when it comes to growing plants, the undisputed majors are water, light and air.
In this article I will discuss a field trial done this year that significantly improved water quality and quantity. How do we increase water in the root zone, and how do we measure this?
First of all, here’s a brief overview of our trial and the results. The site was an irrigated potato field in central Washington State managed by a very talented and successful group of growers. We saw an increase in total soil moisture by over 100 percent in the later part of the potato season and in peak heat. We also observed EC (electrical conductivity) in the root zone and tuber zone varying daily, reaching its lowest point at midday and its highest just prior to midnight, maintaining “ideal” ranges of 0.12 to 0.28 dS/m (deci-siemens per meter).
The treated crop had double the root mass and a massive increase in fine root hairs. We observed no pests even near an economic threshold, and beneficial insects and fungi were abundantly present. There were zero fungicides, nematocides or insecticides applied in season on this crop. It yielded similar to the control, but the cost for nutrient inputs and “crop protection” (poisons) was more than 30 percent less. The soil looked better, smelled better, was less muddy and had better residue decomposition than the control.
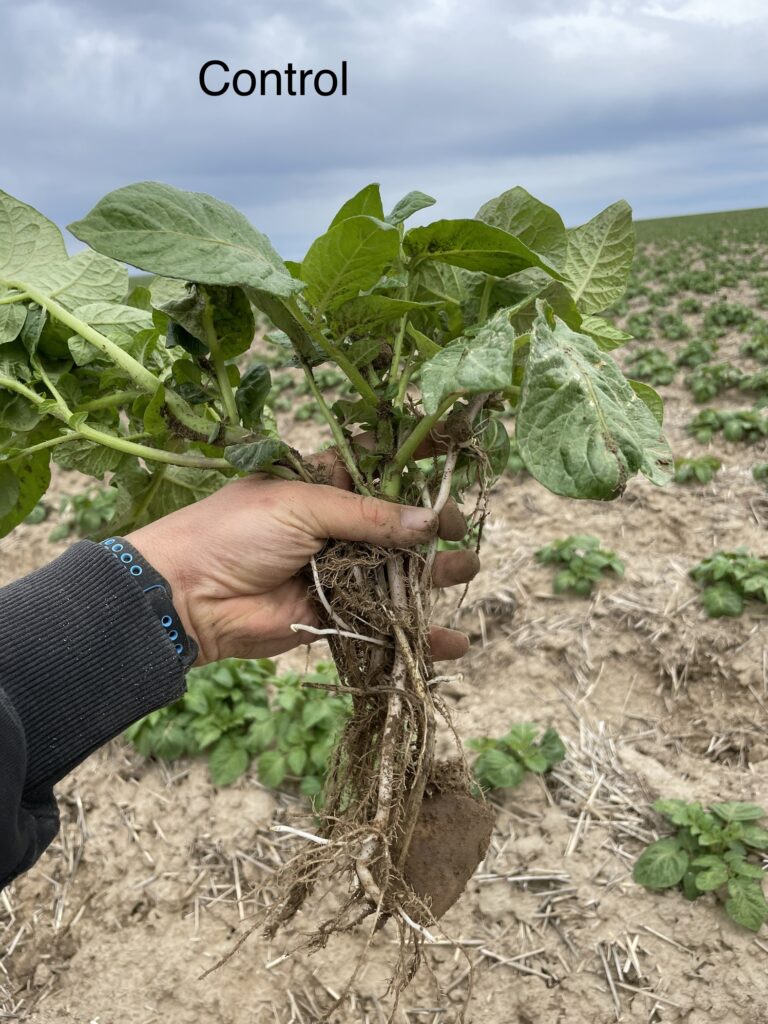
Soil-Plant Water Dynamics
How were we able to get these results? Much of our success was a result of understanding soil-plant water dynamics.
Plants are composed predominantly of water — as much as 95 percent by weight — and their fruit is also incredibly high in water (for example, 80 percent of a potato tuber by weight is water). We also know that plant water content can vary depending on other elements found in the plant sap (e.g., sodium and potassium). TDS (total dissolved solids) also plays a significant role in the plant’s ability to utilize water, since the plant has to parse all the “other stuff” (Na, NO3, K) in the water — some directly absorbable by plant cells and others needing manipulation prior to absorption.
In order to complete the photosynthetic process, water is required to supply an electron via hydrolysis (with manganese required as a cofactor). Water and CO2 — i.e., hydrogen, oxygen and carbon — are the most in-demand elements for plants and life.
So, as way of overview, we know that water is paramount and, in my opinion, doesn’t get the focus it deserves among agronomists. We take this element for granted and become quickly overwhelmed when we take water samples and set off to correct or purify our water sources in irrigation. Rainwater is best, but with today’s pollution levels it comes with its own challenges, including elements like aluminum as well as glyphosate. Rainwater can be very sporadic and can often fall at a rate beyond the infiltration rate and above the holding capacity, causing runoff and the loss of potential yield.
How can we afford the attention, or finance the needed corrections, to such complex and expensive problems? I submit, as a solution, the humble microbe!
The incredible results we achieved came through a crop program based principally on these three principles:
- Apply the highest-quality extract/tea from sources like vermicast and fungal-dominant compost (six applications per season).
- Supply the soil with the purest and highest-quality minerals, like calcium phosphate, calcitic lime and silicon.
- Apply foliars at key development stages, guided by plant-sap tests.
In my experience, the most important of these three principles is the first: the microbial application. I have tried running programs with the minerals and foliars minus our teas and ferments and the results have been mediocre. The invisible workforce is the most impactful aspect when it comes to crop health and productivity, in my experience. The minerals supplied are the building blocks they need, and the foliars are the correction signals sent to steer root exudates for soil mineralization.
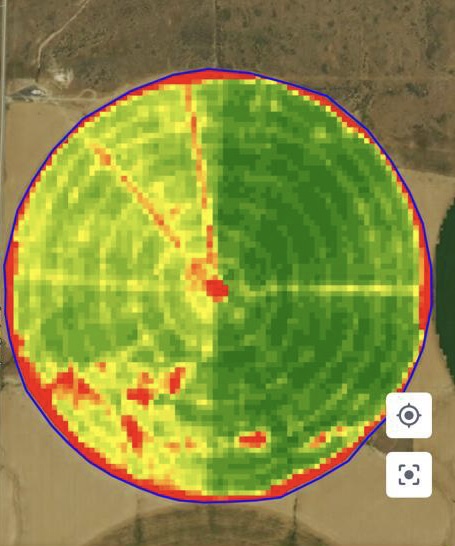
Our Results
The treated half-pivot developed at a similar rate to the control (the control being the grower’s normal program). Initial monitoring of seed pieces for the first eight weeks showed zero rotting. We noticed a significant increase in fine root hair development at ~45 days and continued to observe more fine root hairs the entire season. When scouting for pathogenic fungi and pests, we only observed beneficial white rot of crop residues and beneficial insects like parasitoid wasps, spiders, lacewings, etc.
One of the most surprising observations came near harvest. Our treated side started to show yellowing first, and the NDVI image showed it clearly as well. At first, we were concerned, as the fields usually shut down and began to rot if they yellow early. But in this case, the plants just started showing a bit of yellowing and the plant integrity remained. The vines stayed crisp, with cellular structure intact and no wilting. The grower remarked that it could be a good thing, as hopefully it would actually shuttle nutrients from the vines into the tubers. The yellowing was due to lower vines senescing.
In the control, the vines would rot in the middle — meaning that there was green, wilted growth on either side of the rotten bit in the middle, but not on the treated side. On the treated side, we observed lower vines yellow from the main stem all the way out. The plant released abscisic acid as it should and sacrificed a vine for the better health of the whole plant, and likely reallocated those nutrients to the tuber. That is the way a healthy plant should work! It has an immune system, and when you don’t interrupt it with synthetics, it protects itself.
In our trial next season we will test the tuber to try and determine if the nutrients were reallocated to the tuber as the vines senesce. We will also attempt to test soil percolate to assess water quality and solute elements.
Concentration is all-important. Osmosis and diffusion are dependent on concentration gradients. When you double the water in the same space, the environment changes entirely. The plants and microorganisms are more free to take in what they need. Water-soluble nutrients from synthetic fertilizers are often taken into the plant regardless of need, since they must take in water.
We see this greatly exaggerated in the saps (e.g., Na and Cl) as the C3 photosystem plants off-gas water to cool themselves in the heat. As the water evaporates, the sodium and chloride are left behind to accumulate in the leaves, contributing to exaggerated drought conditions.
Another example is free nitrate that is taken in, and the plant has no choice but to shuttle it into cells with the water, which causes vegetative growth. This can be an even greater problem during reproductive times in plants, when they prefer an ammoniacal form of nitrogen, or when the increased negatively charged water-soluble nitrate fills the cell and displaces other needed anions like phosphate. Dilution affords flexibility and buffers potentially toxic environments. The crop can tolerate higher salt loads and flourish.
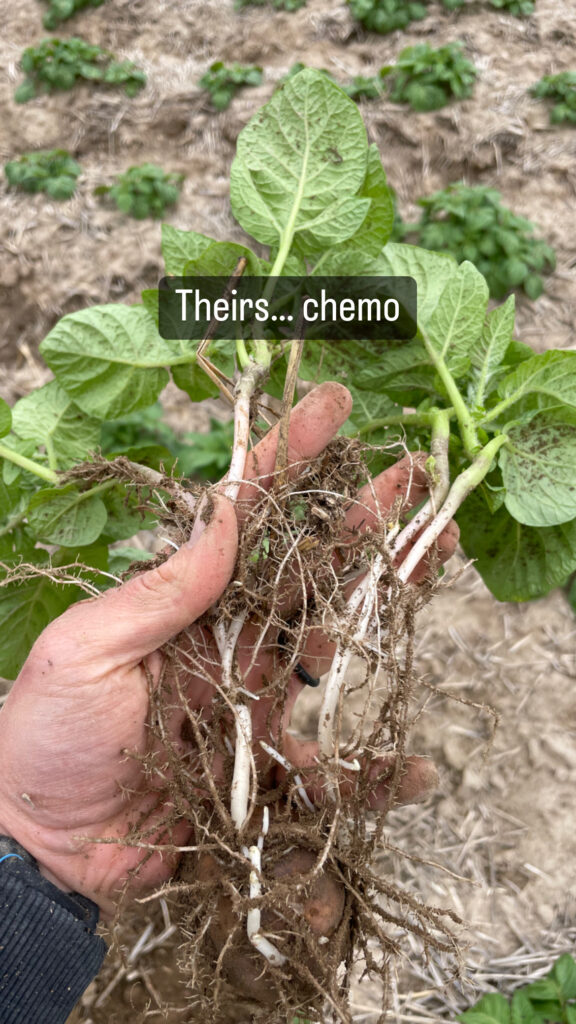
So much of this is undoubtedly attributed to the absence of pesticides. Pesticides influence plants hormonally, negatively impact microbial populations, and change plant root exudates. By supporting the plant naturally through nutrition and microbial partnerships, its immune system is able to function, and the plant physiology will function more as it should.
If you have ever observed soils in the desert and soils in the rainforest, you have probably noticed the difference in plant life but also in fungal populations. In the rainforest, you observe a plethora of fungal hyphae if you peel back the residue on the forest floor. There are many reasons for this, but two of the main ones are the presence of copious residue and humidity/soil moisture. Many soil organisms like AMF will help plants through drought conditions, but all organisms thrive in an abundance of moisture, so long as soils are not saturated and pore spaces are not flooded, starving roots of oxygen.
The treated side of our experiment — the one with over 100 percent more moisture — was not muddy. It had great structure, and even though at times it appeared a bit dry, the moisture sensor indicated higher levels. How can this be? The increase in soil structure, fine root hairs, little microbe bodies, and compounds like glomalin afford more water held tighter, and less mud. It is rather spectacular, especially when you consider we were able to do all this for a significant savings in inputs!
You may be thinking, “this is too good to be true.” We managed to hold the equivalent of roughly more than 430 cubic meters more water. Honestly, the results were much greater than my initial expectations, since this was our first year working with this conventional grower. I was fully prepared to have to intervene with pesticides, as they normally do, but the system responded amazingly to the added biology and supporting nutrients.
As I often say, “life begets life.” When you follow the principles of nature as it is designed, the response is overwhelmingly positive. Creation, within its design and diversity, has the means to correct and purify itself from disastrous conditions! When we observe many conditions in the natural world — volcanoes, flooding, lightning and earthquakes — we see extremophiles prepared to work 24/7 to mitigate the extremes and, over time, transform an unproductive moonscape into a paradise.
If we might humble ourselves and be dedicated to observation and mimicry of the natural world around us, we will see miraculous things. We can see better water quality and produce more with less, leading to abundance and resilience.
Imagine the implications for feeding a growing world population if we could store more than double the water in the root zone in our croplands.
Denver Black is an agronomist with Soilcraft. For a deeper discussion of vermicast tea and water, check out the Regenerative Agronomy podcast.